Trials Evaluating Solarization and Tarping for Improved Stale Seedbed Preparation in the Northeast USA
Abstract:
Stale seedbeds are commonly used by organic vegetable farmers to reduce in-season weed density. The primary purpose of this study was to evaluate the efficacy of soil solarization (clear plastic) with subsequent flaming for stale seedbed preparation. A secondary objective was to compare the efficacy of solarization with tarping (black plastic). Solarization is an established weed management practice in warmer climates, but its efficacy in the humid continental Northeast USA was unknown. We hypothesized that solarization during May-June in Maine, USA would increase weed emergence, and could thereby contribute to depletion of the germinable weed seedbank and, with subsequent flaming, creation of an improved stale seedbed. We expected that firming soil with a roller prior to solarization would further increase weed emergence. Across four site-years of replicated field experiments and two on-farm trials we found that, contrary to expectations, 2 weeks of solarization reduced apparent weed emergence (density) in comparison to nonsolarized controls by 83% during treatment, and 78% after 2 weeks of observation following plastic removal and flaming. Rolling did not significantly affect weed density. Soil temperatures were elevated in solarized plots, reaching a maximum of 47${ }^{\circ} \mathrm{C}$ at 5 cm soil depth, compared to 38${ }^{\circ} \mathrm{C}$ in controls. Weed community analyses suggested that solarization might act as an ecological filter limiting some species. Addressing our secondary objective, two replicated field experiments compared the efficacy of solarization with tarping applied for periods of 2, 4, and 6 weeks beginning in July. Across treatment durations, solarization was more effective than tarping in one site-year, but tarping outperformed solarization in the other; this discrepancy may be explained by differences in weed species and soil temperatures between experiments. Overall, solarization and tarping are promising stale seedbed preparation methods for humid continental climates, but more work is needed to compare their relative efficacy.
1. Introduction
Vegetable growers, including organic growers, commonly use stale seedbed periods prior to sowing high-value crops. Creating a false or stale seedbed, i.e., allowing weeds to emerge and then killing them, often with flaming [1] or shallow cultivation [2], can decrease subsequent weed pressure by depleting the germinable weed seedbank [3]. The use of clear [4] and black plastic mulches [5] to enhance stale seedbed establishment is of interest to organic vegetable farmers in our region, the Northeast USA, many of whom are small to mid-sized growers with abundant weed seedbanks [6], who rely extensively on hand weeding [7].
Soil solarization using clear plastic mulch was developed in the 1970s as a method to control soil borne pathogens [8]. Solarization traps solar radiation, which under suitable conditions elevates soil temperatures enough to cause pest mortality. Its utility as a weed control technique in arid and some Mediterranean regions is well documented [9–11]; however, solarization has received less research attention in cooler regions. Solarization in Oregon, USA reduced Poa annua L. seed viability by 89 to 100% at depths less than 5 cm [12], and six weeks of solarization reduced weed density by 38 to 54% but did not improve strawberry yield in Virginia, USA [13]. Covering soil with perforated polyethylene tarps in England, UK increased weed emergence [14]. Studies testing solarization for control of fungal pathogens in the Northwest USA and Canada have shown mixed results [15,16], leading to the conclusion in a review by Walters and Pinkerton [17] that solarization is not consistently effective in cool northern regions. Therefore, we expected that solarization alone during the springtime in Maine would not cause weed mortality, but would instead deplete the weed seedbank by increasing weed emergence, allowing weeds to be killed with subsequent flaming.
Tarping, also known as occultation [5], is the practice of using black plastic silage tarps applied to the soil for several weeks prior to planting as a method for stale seedbed preparation. It is closely related to the practice of anaerobic soil disinfestation [18] but is not intended to result in prolonged anaerobic soil conditions. Tarping can decrease subsequent weed seed germination [19], but it is not always effective [20,21]. The few studies that have compared solarization and tarping for weed control suggest that solarization is usually more effective than tarping [20,22,23], likely due to higher soil temperatures achieved under solarization [24].
However, in one study conducted during the fall in Israel, tarping outperformed solarization [25] perhaps because soil temperature during this relatively cool season was insufficient for weed control via solarization. In the Northeast USA, a single-year study found that tarping outperformed solarization as a method of cover crop termination [26], but we are aware of no prior studies comparing solarization and tarping for stale seedbed establishment in our region.
The primary objective of this study was to test whether solarization combined with flaming could improve the efficacy of stale seedbed establishment in the Northeast USA. A secondary objective was to compare the weed control efficacy of solarization to tarping. Field experiments were conducted in 2015-2017 to test the following hypotheses:
1. Springtime soil solarization will increase weed emergence;
2. Firming soil with a roller will further increase weed emergence;
3. The seedbank depletion resulting from solarization and rolling will reduce weed emergence in a subsequent stale seedbed created by flaming; and
4. During mid-summer, solarization will be more effective than tarping for stale seedbed establishment.
2. Materials and Methods
To test Hypotheses 1 to 3, replicated field experiments were conducted over four site-years near Orono, Maine, USA (Table 1; Figure A1). Additional data were collected during two on-farm demonstrations in Winthrop and Harborside, Maine, USA in May to June of 2015. The monthly 30-year climate averages for the period were 14.7 ${ }^{\circ} \mathrm{C}$ mean temperature and 9.4 cm precipitation [27].
Site-year | Location | Soils | Dates | ||
Rogers 2015 | 44$^{\circ}$55’N 68$^{\circ}$41’W | Pushaw-Boothbay complex; 4.6% OM and 6.4 pH (2011) | 16.0 | 15.9 | solarization: 27 May – 12 June observation: 12 June – 30 June |
UMG 2015 | 44$^{\circ}$54’N 68$^{\circ}$39’W | Peru-Tunbridge association; 6.6% OM and 6.1 pH (2012) | 15.0 | 14.4 | solarization: 15 May – 3 June observation: 3 June – 22 June |
Rogers 2016 | 44$^{\circ}$55’N 68$^{\circ}$41’W | Pushaw-Boothbay complex; 3.7% OM and 6.2 pH (2014) | 16.4 | 7.7 | solarization: 13 May – 31 May observation: 31 May – 14 June |
Smith 2016 | 44$^{\circ}$54’N 68$^{\circ}$41’W | Nicholville very fine sandy loam; 5.0% OM and 5.9 pH (2014) | 16.9 | 6.3 | solarization: 18 May – 1 June observation: 1 June – 15 June |
Field experiments included four treatments, arranged in a randomized complete block design with three replications per site-year (Figure A2). Treatments included:
• Tilled (control)
• Tilled + rolled (control)
• Tilled + solarized
• Tilled + rolled + solarized
Prior to establishment of each experiment, soils were rototilled to 15 cm soil depth, except for the Smith 2016 experiment in which the field was moldboard ploughed followed by cultivation with a Perfecta field cultivator (Unverferth Manufacturing Co., Inc., Kalida, Ohio, USA). In all experiments a 45.4 kg lawn roller was used to simulate cultipacking. This tool was appropriate to the scale of these experiments, but likely firmed soil more consistently than would a standard ring cultipacker. Prior to mulching, all plots were irrigated to approximate field capacity to increase heat conduction [30]. Solarized treatments were covered with previously used 6-mil clear polyethylene film (hereafter referred to as plastic), salvaged from two greenhouses on the University of Maine campus. Holes in the plastic were minimal, and were patched with tape when present. Previously used greenhouse plastic was chosen to represent likely grower management practices for our region. Plastic from the same source was used within blocks.
Plots were 3 m by 3 m with 0.6 m between plots. To secure plastic while keeping plots accessible for measurement during treatment, plastic edges were clipped to 3.3 cm diameter by 3.2 m long pieces of galvanized metal pipe laid in 10 cm deep trenches around plot perimeters. Plastic was removed after approximately two weeks of solarization (Table 1), after which stale seedbeds were prepared with no further soil disturbance by flaming all plots using a handheld single burner propane torch, moving the end of the nozzle over the field at a height of 10 cm and a speed of 0.25 m s-1. The effect of flaming was measured during the Rogers 2015 site-year by employing a split-plot design with presence/absence of flaming as subplot treatments. Two on-farm trials conducted in the spring of 2015 each consisted of a single replicate of the tilled and tilled + solarized treatments (Figure A2), following standard protocols.
Soil temperatures were logged hourly for the duration of solarization treatment using iButton temperature loggers (Maxim Integrated, San Jose, California, USA). One logger per plot was placed in a sealed 5 cm by 5 cm 4-mil plastic bag and buried at 5 cm soil depth. Volumetric soil moisture content was measured and averaged across three locations within each plot using a Delta-T soil moisture meter (HH2 version 4.0, Delta-T Devices Ltd, Cambridge, England) at the start of each experiment and concurrent with each weed censuses (described below).
Weeds were counted once every 2 to 7 days during solarization treatment, and approximately every 7 days for 2 weeks following solarization. Plastic was temporarily removed during census counts. During each census, weed seedlings were counted and pulled from permanent 0.25 m by 0.5 m quadrats during the solarization period, and from a new set of permanent quadrats during the period following solarization. The four weed taxa most abundant in each quadrat were identified and counted; remaining weeds were counted as other broadleaved or other grass-like. Weeds were identified to species level with the following exceptions: Lolium spp. and Gnaphalium spp. were identified to genus, and members of the Brassicaceae other than Capsella bursa-pastoris L. Medik. (likely Brassica and Rorippa spp.) were grouped as other brassicas. If few weeds were present, additional quadrats were added consecutively to the right of permanent quadrats and counts summed until$\geq$25 total weeds were counted or four quadrats sampled, whichever occurred first. Counts were adjusted for effective quadrat size, and summed to account for differences in number of censuses conducted at different site-years. Data representing weed emergence are thus reported as cumulative weed density m-2 in each plot during solarization and after solarization, respectively.
All analyses were performed in R [31]. Mixed effects models were constructed using the nlme package [32], means separations performed using the multcomp package [33], linear discriminant analyses performed using the MASS package [34] and other multivariate analyses performed using the vegan package [35]. Response variables were square root transformed prior to analysis to improve normality and homogeneity of variances; statistical assumptions were met unless otherwise indicated below. The chosen significance level was $\alpha$ = 0.05.
To determine whether solarized and rolled treatment effects were significant across site-years, we fit linear mixed effects (LME) models to the weed density data from all four site-years of experiments (Table 1) plus two on-farm trials. These models were chosen in part because they are appropriate for unbalanced designs [36]. Separate models were fit for the period during solarization, and the observation period after solarization, with cumulative weed density m-2 as the response, treatment as a fixed effect, and site-year as a random effect. Means were separated by Fisher’s Protected LSD. The effects of flaming on solarization efficacy were tested using analysis of variance (ANOVA) and pre-planned contrasts.
To test for treatment effects on weed community composition, permutational multiple analysis of variance (PERMANOVA) models were fit for the period during solarization and the period after solarization, respectively, using Euclidean distances and 999 permutations [37]. PERMDISP tests were performed using Euclidean distances and 999 permutations to test for homogenous dispersion among groups [37]. These methods were selected because the data were not multivariate normal. Species observed in fewer than 10% of plots were dropped prior to analyses. The effects of treatment on the weed community were further explored through linear discriminant analyses (LDA) [38]. Separate analyses were conducted for the period during solarization and the period following solarization, with linear discriminant functions first constructed to discriminate weed communities by treatment. Classification using jackknifed discrimination matrices suggested these functions discriminated poorly, correctly classifying data in 36% and 29% of instances, respectively, for the periods during and after solarization, compared with 25% correct expected based on randomness. Because most misclassifications resulted from a poor ability of the functions to discriminate based on rolling, a second set of functions was created to discriminate between data pooled as solarized and nonsolarized. These performed better, correctly classifying in 92% and 64% of instances, respectively, for the periods during and after solarization, with 50% correct expected based on randomness.
To compare solarization to tarping, experiments (hereafter TARP) were conducted at the University of Maine Rogers Farm $\left(44^{\circ} 55^{\prime} \mathrm{N}, 68^{\circ} 41^{\prime} \mathrm{W}\right)$ in July to September of 2016 and 2017. Soils were Pushaw-Boothbay complex [28] in both fields (Figure A3). The 2016 field had pH of 6.2 and 3.7% organic matter (2014 soil test); the 2017 field had pH of 5.8 and 3.0% organic matter (2017 soil test). The monthly 30-year climate averages for the period were 8.7 cm precipitation and 18.2 ${ }^{\circ} \mathrm{C}$ mean temperature [27]. During the eight week experimental periods, the mean air temperature and total precipitation were, respectively, 21.8 ${ }^{\circ} \mathrm{C}$ and 8.0 cm in 2016; 19.0 ${ }^{\circ} \mathrm{C}$ and 11.4 cm in 2017 [29].
Experiments consisted of seven treatments arranged in a randomized complete block design with three replications. Six mulched treatments consisted of factorial combinations of plastic mulch (solarization, tarping) and treatment duration (2, 4, and 6 weeks); the seventh treatment was a nonmulched control. Plots were 1 m by 1 m with 0.6 m between plots, which was considered the minimum size needed to avoid strong edge effects [39]. The field was rototilled to 15 cm depth prior to experiment start dates, and irrigated prior to mulching. Solarization plots were covered with salvaged 6-mil clear polyethylene greenhouse plastic; tarping plots with 3-mil black plastic silage tarp (Belson Experiments consisted of seven treatments arranged in a randomized complete block design with three replications. Six mulched treatments consisted of factorial combinations of plastic mulch (solarization, tarping) and treatment duration (2, 4, and 6 weeks); the seventh treatment was a nonmulched control. Plots were 1 m by 1 m with 0.6 m between plots, which was considered the minimum size needed to avoid strong edge effects [39]. The field was rototilled to 15 cm depth prior to experiment start dates, and irrigated prior to mulching. Solarization plots were covered with salvaged 6-mil clear polyethylene greenhouse plastic; tarping plots with 3-mil black plastic silage tarp (Belson
Following the methods detailed in section 2.1.3., soil temperature was logged hourly at 5 cm soil depth during treatment, and volumetric soil moisture measured prior to mulching and concurrent with weed censuses. Weeds were counted on days mulch treatment was terminated, and approximately 14 days after termination of each respective treatment. In 2016, census dates were 9 August, 23 August, and 9 September; in 2017, censuses dates were 22 August, 7 September, and 20 September. Censuses were performed in single 0.25 m by 0.5 m permanent quadrats located in the center of each plot. Control plots were censused concurrently with each mulch treatment census; to accommodate this design, weeds were not pulled during census counts. In 2016, weeds were identified as either broadleaved or grass-like. In 2017, weeds were counted by taxa following the methods in section 2.1.3.
The nonmulched control treatment was excluded from statistical analysis due to pseudoreplication in the experimental design and because this treatment was not essential to our objective of comparing solarization and tarping efficacy. Data were analyzed in R [31], with response variables square root or log10 +1 transformed as necessary to meet assumptions. Initial ANCOVA models suggested significant year effects, so years were analyzed separately. In both years, weed density was zero in tarped plots of any duration at plastic termination. To test whether weed density in solarized plots significantly exceeded these zero values, one-sided Welch’s t-tests were performed for data pooled across treatment durations. Data from weed censuses performed 14 days after plastic termination were analyzed using ANCOVA with weed density as the response, and explanatory variables mulch treatment, duration (numeric), and treatment by duration interaction. Following ANCOVA, means were separated by Fisher’s protected LSD using the multcomp package [33] in R [31].
3. Results
In our spring experiments, soil temperatures were elevated under solarization, with maximum temperatures ranging from 32 to 47${ }^{\circ} \mathrm{C}$ at a depth of 5 cm in solarized plots, as compared with 29 to 38${ }^{\circ} \mathrm{C}$ in controls. Soil moisture was greater in rolled treatments (Table 2). During treatment, there was 83% and 81% less weed density in tilled + solarized and tilled + rolled + solarized treatments, respectively, as compared with corresponding controls (Figure 1A). During 14 days of observation following plastic termination, weed density was 78% and 75% less in tilled + solarized and tilled + rolled + solarized treatments, respectively, as compared with controls (Figure 1B). These treatment effects were reasonably consistent across site-years both during $\left(\mathrm{R}_{\text {marginal }}^2=0.43, \mathrm{R}_{\text {conditional }}^2=0.73, \mathrm{X}^2=81, p<0.01\right)$ and after solarization $\left(R_{\text {marqinal }}^2=0.28, \mathrm{R}^2\right.$ conditional $=$ $\left.0.39, X^2=23, p<0.01\right)$.
There was no significant difference in weed density between flamed and nonflamed subplots in either solarization treatment (tilled + solarized: t = -0.49, p = 0.63; tilled + rolled + solarized: t = -1.09, p = 0.29). Flaming significantly reduced weed density in the tilled control treatment (t = -2.85, p = 0.01), and caused a 32% reduction in weed density in the tilled + rolled treatment, though this difference was not statistically significant (t = -1.05, p = 0.31).
PERMANOVA models suggested non-significant effects of treatment on weed community composition during (R2 = 0.09, F3;48df = 1.66, p = 0.09), and after solarization (R2 = 0.09, F3;48df = 1.67, p = 0.06). Significant PERMDISP tests for the periods during (F3;48df = 5.84, p <0.01) and after solarization (F3;48df = 6.76, p <0.01) indicated differences in dispersion (beta diversity) between treatment groups [37]. Linear discriminant analyses showed clear separation between solarized treatments and control treatments along the first linear discriminant function during the solarization period (Figure 2A). Rolling appeared to drive separation between control treatments but not solarized treatments during the period after solarization (Figure 2B). All weed species decreased in abundance under solarization (data not shown); however, LDA coefficients (Table 3) suggested that winter annuals (Capsella bursa-pastoris; Stellaria media (L.) Vill.; Poa annua) and Trifolium repens L. were disproportionately reduced during the solarization period, as compared with other species, and Poa annua remained disproportionately reduced after solarization.


Treatment | Soil temperature | Exposure time (h) | Soil moisture (%vol) | ||||
Maximum | Average | $36-40^{\circ} \mathrm{C}$ | $41-45^{\circ} \mathrm{C}$ | $>45^{\circ} \mathrm{C}$ | Start | End | |
Tilled control | 32 ± 2 | 17 ± 1 | $<1$ | 0 | 0 | 21 ± 8 | 13 ± 3 |
Tilled + rolled control | 32 ± 2 | 17 ± 1 | 0 | 0 | 0 | 29 ± 7 | 20 ± 4 |
Tilled + solarized | 42 ± 4 | 24 ± 2 | 21 ± 12 | 12 ± 11 | $<1$ | 21 ± 7 | 13 ± 4 |
Tilled + rolled + solarized | 42 ± 3 | 23 ± 3 | 20 ± 11 | 12 ± 12 | $<1$ | 27 ± 7 | 20 ± 4 |
Weed species | Common name | Eigenvectors | |
During solarization | After solarization | ||
Elymus repens | quackgrass | 0.12 | -0.03 |
Amaranthus retroflexus | redroot pigweed | -0.04 | -0.06 |
Ambrosia artemisifolia | common ragweed | -0.15 | |
Capsella bursa-pastoris | shepherd's-purse | -0.47 | -0.06 |
Chenopodium album | common lambsquarters | -0.16 | 0.02 |
Digitaria sanguinalis | large crabgrass | 0.04 | -0.07 |
Echinochloa crus-galli | barnyardgrass | 0.06 | -0.06 |
Galinsoga quadriradiata | hairy galinsoga | -0.06 | -0.01 |
Panicum capillare | witchgrass | -0.08 | |
Poa annua | annual bluegrass | -0.30 | -0.31 |
Portulaca oleracea | common purslane | -0.13 | |
Stellaria media | common chickweed | -0.36 | |
Trifolium repens | white clover | -0.37 |
Solarization resulted in higher maximum and average soil temperatures than did tarping (Table 4). In both years of our TARP study, weed density was zero at plastic termination in tarping treatments (Table 5). In 2016, weed density at termination of solarization treatments was very low and, across treatment durations, not significantly different than zero (Table 5; t = 2.29, p = 0.05). In 2017, weed emergence (density) was significant during solarization (Table 5; t = 6.00, p <0.01). Our ANCOVA model for weed density following plastic termination in 2016 (R2 = 0.80) included significant effects for treatment, duration, and treatment by duration interaction; specifically, solarization resulted in less subsequent weed density than tarping and was more effective with increasing treatment duration (Table 5), while tarping efficacy was lowest following 4 weeks of treatment. The corresponding model for 2017 (R2 = 0.83) included significant effects for treatment only, with greater weed density following solarization than tarping (Table 5). Density of the most abundant species in our 2017 study system, Portulaca oleracea L., was higher in solarized plots than controls, while density of other broadleaved weeds was reduced by solarization (Figure 3).

Year | Treatment | Duration (weeks) | Soil temperature | Exposure time (h) | Soil moisture (%vol) | ||||
Maximum | Average | $36-40^{\circ} \mathrm{C}$ | $41-45^{\circ} \mathrm{C}$ | $>45^{\circ} \mathrm{C}$ | Start | End | |||
2016 | control | 2 | 35 ± 1 | 24 ± 0 | $<1$ | 0 | 0 | 36 ± 6 | 12 ± 1 |
4 | 35 ± 1 | 23 ± 0 | $<1$ | 0 | 0 | 36 ± 6 | 10 ± 2 | ||
6 | $\mathrm{nd}^{\dagger}$ | nd | nd | nd | nd | 36 ± 6 | 23 ± 2 | ||
tarping | 2 | 41 ± 2 | 28 ± 0 | 28 ± 11 | 3 ± 3 | 0 | 35 ± 5 | 18 ± 2 | |
4 | 41 ± 2 | 28 ± 0 | 71 ± 17 | 8 ± 12 | 0 | 38 ± 2 | 18 ± 2 | ||
6 | 41 ± 3 | 27 ± 0 | 72 ± 55 | 11 ± 10 | 0 | 36 ± 5 | 17 ± 1 | ||
solarization | 2 | 46 ± 3 | 31 ± 1 | 48 ± 11 | 30 ± 21 | 4 ± 8 | 33 ± 5 | 18 ± 3 | |
4 | 46 ± 3 | 31 ± 1 | 101 ± 12 | 30 ± 21 | 8 ± 11 | 33 ± 1 | 16 ± 3 | ||
6 | $50 \pm 1^{\ddagger}$ | $31 \pm 0 \ddagger$ | $108 \pm 2^{\ddagger}$ | $117 \pm 2^{\ddagger}$ | $49 \pm 8^{\ddagger}$ | 39 ± 3 | 17 ± 5 | ||
2017 | control | 2 | $33 \pm 4 \ddagger$ | $23 \pm 1 \ddagger$ | $0^{\ddagger}$ | $0^{\ddagger}$ | $0^{\ddagger}$ | 22 ± 3 | 7 ± 1 |
4 | $33 \pm 4 \ddagger$ | $22 \pm 1^{\ddagger}$ | $0^{\ddagger}$ | $0^{\ddagger}$ | $0^{\ddagger}$ | 22 ± 3 | 10 ± 1 | ||
6 | $33 \pm 4 \ddagger$ | $21 \pm 1^{\ddagger}$ | $0^{\ddagger}$ | $0^{\ddagger}$ | $0^{\ddagger}$ | 22 ± 3 | 33 ± 4 | ||
tarping | 2 | $39 \pm 1^{\ddagger}$ | $25 \pm 0^{\ddagger}$ | $16 \pm 7^{\ddagger}$ | $0^{\ddagger}$ | $0^{\ddagger}$ | 30 ± 4 | 12 ± 0 | |
4 | $37 \pm 0^{\ddagger}$ | $25 \pm 0^{\ddagger}$ | $12 \pm 1^{\ddagger}$ | $0^{\ddagger}$ | $0^{\ddagger}$ | 26 ± 5 | 15 ± 2 | ||
6 | 39 ± 1 | 24 ± 0 | 31 ± 18 | 1 ± 2 | 0 | 29 ± 4 | 28 ± 6 | ||
solarization | 2 | $46 \pm 0^{\ddagger}$ | $29 \pm 1^{\ddagger}$ | $32 \pm 8^{\ddagger}$ | $33 \pm 6^{\ddagger}$ | $5 \pm 1^{\ddagger}$ | 29 ± 11 | 13 ± 5 | |
4 | 43 ± 3 | 27 ± 1 | 40 ± 14 | 17 ± 16 | $<1$ | 32 ± 6 | 14 ± 1 | ||
6 | 45 ± 1 | 27 ± 1 | 51 ± 36 | 25 ± 6 | 1 ± 2 | 30 ± 2 | 28 ± 11 |
Year | Treatment | Duration (weeks) | Total weed density (no m ) | |
Termination | Termination +14 | |||
2016 | control | 2 | 595 ± 114 | 803 ± 127 |
4 | 803 ± 127 | 635 ± 46 | ||
6 | 635 ± 46 | 680 ± 44 | ||
tarping | 2 | 0 | 261 ± 67 | |
4 | 0 | 640 ± 130 | ||
6 | 0 | 205 ± 69 | ||
solarization | 2 | 5 ± 5 | 141 ± 14 | |
4 | 11 ± 7 | 16 ± 5 | ||
6 | 5 ± 5 | 11 ± 5 | ||
2017 | control | 2 | 56 ± 12 | 403 ± 101 |
4 | 403 ± 101 | 320 ± 47 | ||
6 | 320 ± 47 | 453 ± 107 | ||
tarping | 2 | 0 | 32 ± 9 | |
4 | 0 | 0 | ||
6 | 0 | 27 ± 16 | ||
solarization | 2 | 419 ± 134 | 427 ± 130 | |
4 | 571 ± 50 | 288 ± 41 | ||
6 | 224 ± 61 | 237 ± 80 |
4. Discussion
Contrary to expectations (Hypothesis 1), but nevertheless a desirable weed management outcome, springtime soil solarization greatly reduced weed density during two weeks of treatment (Figure 1A). The weed-suppressive effect of solarization persisted after plastic was removed and plots were flamed. There was a trend toward increased weed density in rolled treatments, as expected (Hypothesis 2), but the magnitude of the solarization effect was greater and differences based on rolling were not significant (Figure 1). The finding that nonflamed subplots were not weedier than flamed subplots suggests that two weeks of solarization alone can create an excellent stale seedbed in our region, the Northeast USA.
The maximum temperatures and accumulated time under high temperature conditions measured at 5 cm depth during these experiments (Table 2) are less than published thresholds required for weed seed mortality in some species [40,41]. However, higher maximum temperatures were likely reached nearer the soil surface [42,43]; data from our own methods development indicates that maximum temperatures may have been $\geq 5^{\circ} \mathrm{C}$ greater at 1 cm as compared with 5 cm soil depth (Birthisel SK, unpublished data). Further, we observed dead white-thread stage weeds under the solarization plastic in some plots. These were not accounted for in our weed censuses, but their presence suggests that germination and subsequent seedling death was a mechanism of seedbank reduction in these experiments. The pattern in our weed community data following solarization (Figure 2B), along with PERMDISP test results, suggests that solarization reduced beta diversity, or in-group dispersion, in comparison with control treatments. This is consistent with the hypothesis and findings of Chase [44] and suggests that solarization can act as a filter shaping weed community composition [45]. Though winter annuals and Poa annua were disproportionately harmed during solarization (Table 3), the contributions of other weed species to the overall community were weakly impacted by solarization, and none strongly positively associated (Table 3). This suggests that solarization can be effective against many weeds present in the Northeast USA, consistent with Cohen and Rubin’s review of species susceptibility to solarization [9]. Two susceptible weeds in particular, Galinsoga quadriradiata (Raf.) Blake and Digitaria sanguinalis (L.) Scop. [41], are among the most problematic for regional organic farmers [6].
Our decision to employ previously used greenhouse plastic in these experiments may have impacted results. There is a considerable body of research characterizing the effects of plastic optical properties on soil heating [39], and specialized mulches designed to optimize efficacy have been tested [46–48]. We lacked the resources to quantify optical characteristics of the polyethylene used in these experiments. However, studies comparing the use of new and previously used polyethylene for solarization suggest that previously used polyethylene can work as well or better than new [46,49], so we do not necessarily expect that efficacy was diminished. Specialized solarization films [50] or modifications such as the use of bubble film for solarization [51] could perhaps improve efficacy, though the increased soil heating from the use of specialized films does not always translate to improved weed control outcomes [46,52].
Solarization, which has long been of interest as a relatively environmentally-friendly alternative to chemical fumigants [8], warrants further study as a promising strategy for improving stale seedbed preparation on organic farms in the Northeast USA. We hope future work in our region will measure the impact of solarization on weed seedbank depletion [3], assessing it’s potential to cause long-term reductions in weed pressure. Growers in our region have asked whether the in-season weed control benefits of solarization offset labor and opportunity costs, resulting in economic returns. Solarization was economically advantageous in California strawberries [53]; however, a study in California organic vegetables found that flame weeding was more cost-effective [54]. An economic assessment specific to small and midsized vegetable growers in the Northeast USA could aid in the creation of local farm management recommendations. Growers have also asked about the impacts of solarization in the Northeast USA on beneficial soil microbiota, which we address elsewhere (Birthisel et al., this issue).
We had expected solarization to result in higher soil temperatures and better weed control outcomes than tarping (Hypothesis 4). Results of our 2016 TARP experiment (Table 4; Table 5) support this hypothesis, corroborating a majority of published experiments on the topic [55]. In our 2017 experiment, however, tarping was more effective than solarization (Table 5). The abundance of Portulaca oleracea in our 2017 study site was likely an important factor influencing this result; emergence of this species was apparently promoted by solarization (Figure 3). Consistent with this finding, Dahlquist et al. [40] report that P. oleracea readily germinated at temperatures of 42 and 46 ${ }^{\circ} \mathrm{C}$. Though we did not collect weed species data at the plot level in 2016, when solarization proved more effective (Table 5), we noted that the four most abundant species in the field were Amaranthus retroflexus, Chenopodium album, Galinsoga quadriradiata, and Echinochloa crus-galli, all annuals that were well controlled in our other experiments (Figure 3; Table 3). Another factor that may have contributed to the discrepancy in results between site-years is soil temperature: overall, hotter temperatures and greater accumulations of time at high temperatures were measured in 2016 than in 2017 (Table 4).
We had expected the efficacy of both solarization and tarping to increase with treatment duration, but our data offer weak and inconsistent support for this idea. Treatment duration was not a significant factor in 2017. In 2016, solarization efficacy did increase with treatment duration (Table 5). However, weed density following tarping was unexpectedly 146% greater in the 4 week treatment as compared with the 2 week treatment (Table 5). The timing of rainfall during this atypically dry summer may explain this result: 43 mm of rain fell during the period of observation following plastic removal in the 4 week treatments, whereas only 13 mm and 8 mm of rain fell during the observation periods following the 2 week and 6 week treatments, respectively [29]. Since moisture cues are typically required for germination [56] and weeds at the seedling stage may be especially sensitive to desiccation, greater density might have been expected following 2 weeks of tarping if conditions had been more favorable to germination and establishment. The fact that weed emergence did not appear to be stimulated by rainfall in the 4 week solarization treatment (Table 5) suggests that this treatment may have been effective in depleting the germinable weed seedbank.
Overall, these results suggest tradeoffs between solarization and tarping that should be more thoroughly characterized before either strategy is advocated as a “better” approach for farmers in the Northeast USA and areas of similar climate. Solarization applied as a stale seedbed technique to susceptible species under good conditions may result in greater seedbank depletion than tarping [19] thereby offering longer-term benefits. However, the light blocking effect of tarping may make it more suitable under marginal conditions, or in situations where the intended purpose is simply to prevent weed emergence for a period of time rather deplete the seedbank. Research comparing these practices over a wider range of soil, weather, and seedbank conditions could aid in the development of guidelines to help growers select practices that align with their situations and goals. We advocate as well that further studies follow Lounsbury et al. [26] in examining the utility of solarization and tarping for terminating cover crops prior to organic no-till or strip-till plantings. Recent work on ‘biosolarization’ [57] and anaerobic soil disinfestation indicates that incorporation of crop residues [58] and other organic amendments [42,59] prior to treatment can increase the weed control efficacy of solarization and tarping. Given farmer interest in organic reduced tillage, this could be a fruitful area for future work.
5. Conclusion
Across replicated experiments and on-farm trials, two weeks of springtime soil solarization followed by flaming created a stale seedbed with 78% less subsequent weed density than a control stale seedbed prepared with flaming only. Nonflamed subplots established during one site-year suggested that solarization alone, without flaming, can create an effective stale seedbed. Soil temperatures measured under solarization may have contributed to thermal inactivation of some species of weed seed, and fatal germination of others. Multivariate weed community analyses indicate that solarization may act as an ecological filter shaping weed community composition. We hope future studies of solarization will more thoroughly characterize its impacts on weed seedbanks, and evaluate whether the practice is economically advantageous to growers in our region. Additional experiments compared the efficacy of solarization to tarping with black plastic. Solarization outperformed tarping in one year of study, but the opposite was true the following year. Higher temperatures in our first year experiment, and high density of the relatively heat-tolerant weed Portulaca oleracea (purslane) in our second, may explain these discrepant results. Overall, solarization and tarping are promising organic stale seedbed preparation techniques, but more work is needed to evaluate their relative efficacy over a range of conditions and applications relevant to growers in humid continental climates like the Northeast USA.
Thanks to Craig Hickman for the excellent question that inspired this project, to Eliot Coleman for his deep knowledge, and to both for collaborating in on-farm trials. We greatly appreciate the excellent work of Liam Kenefic and our other field crew members: Ana Eliza Souza Cunha, Grace Smith, Lucia Helder, and Anthony Codega. Thanks also to Brad Libby, Joe Cannon, Laureen Traclet, Tom Molloy, Tamara Levitsky, Corianne Tatariw, Mark Guzzi, Margaret McCollough, Bryan Brown, Avinash Rude, Brian McGill, Ellen Mallory, Jay Hao, David Hiebeler, our anonymous reviewers, and the many farmers whose questions and insights helped guide this project. This work was made possible by funding from the Maine Food and Agriculture Center and the University of Maine Graduate Student Government. This project was further supported by the USDA National Institute for Food and Agriculture, Hatch Project Number ME0-21606 through the Maine Agricultural and Forest Experiment Station. This is MAFES publication number 3705.
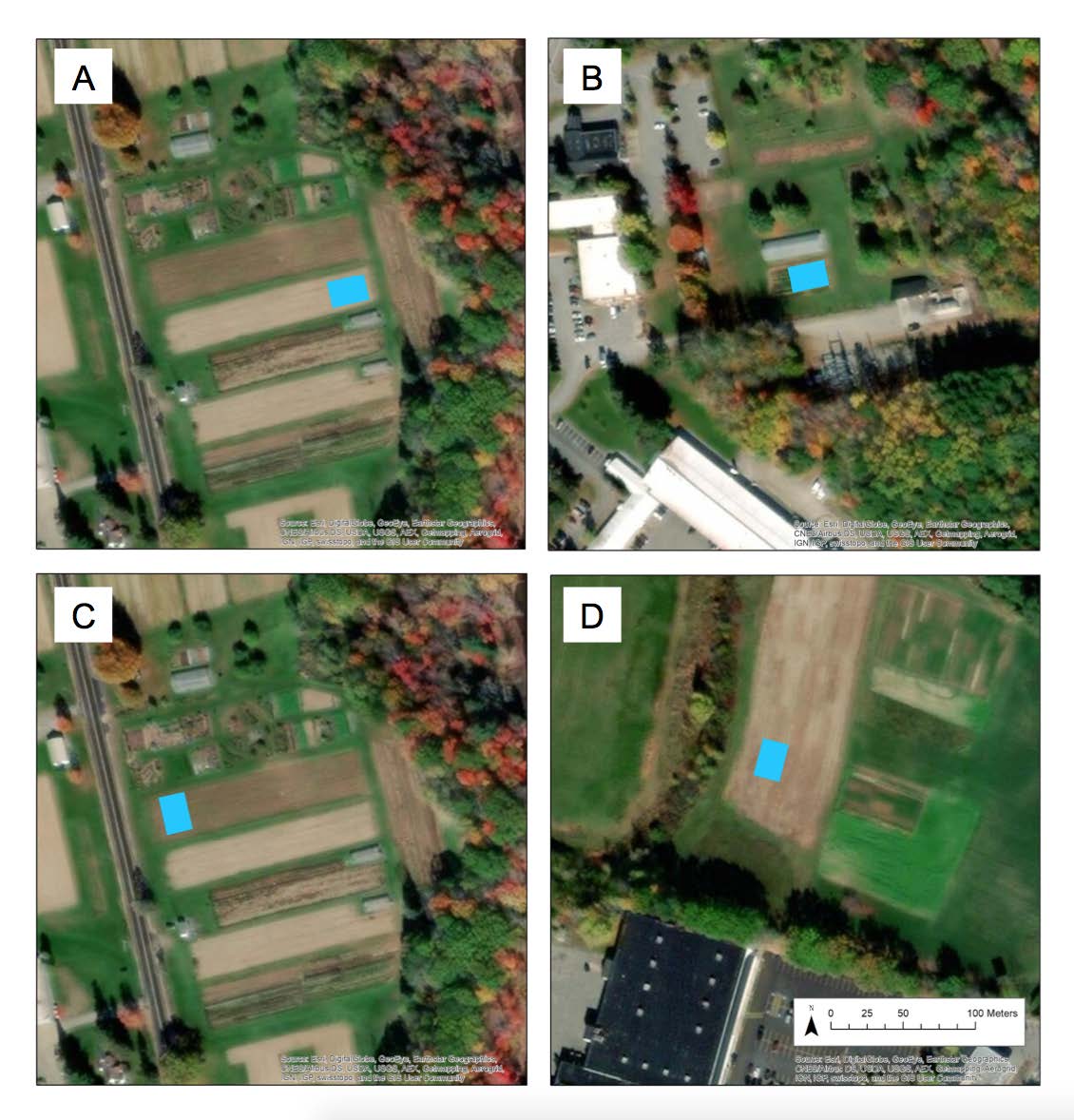
Figure A1. Aerial view showing locations of four spring solarization experiments: (A) Rogers 2015, (B) UMG 2015, (C) Rogers 2016, and (D) Smith 2016
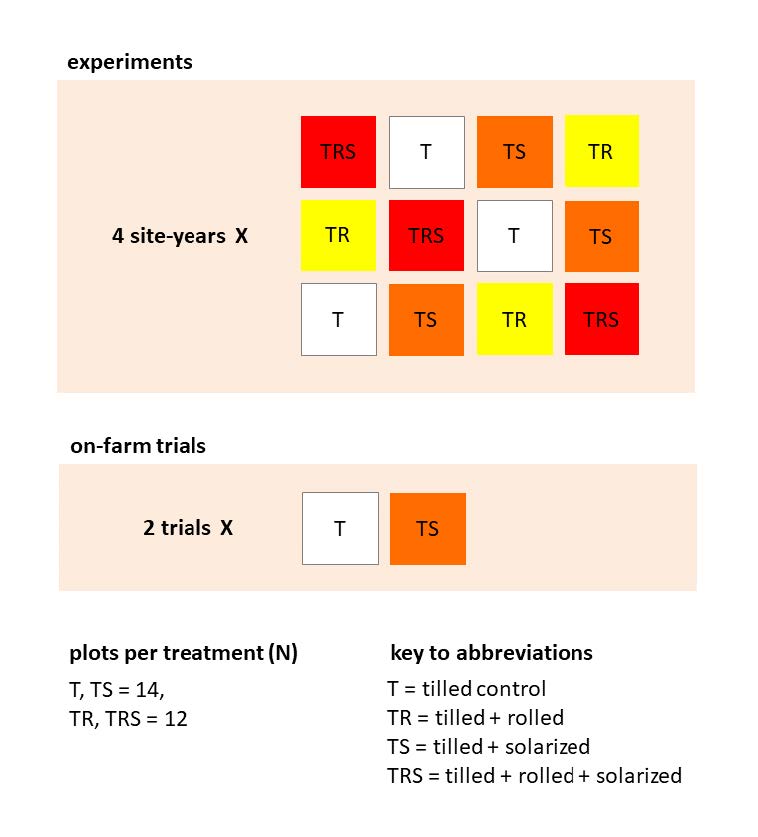
Figure A1. Aerial view showing locations of four spring solarization experiments: (A) Rogers 2015, (B) UMG 2015, (C) Rogers 2016, and (D) Smith 2016.
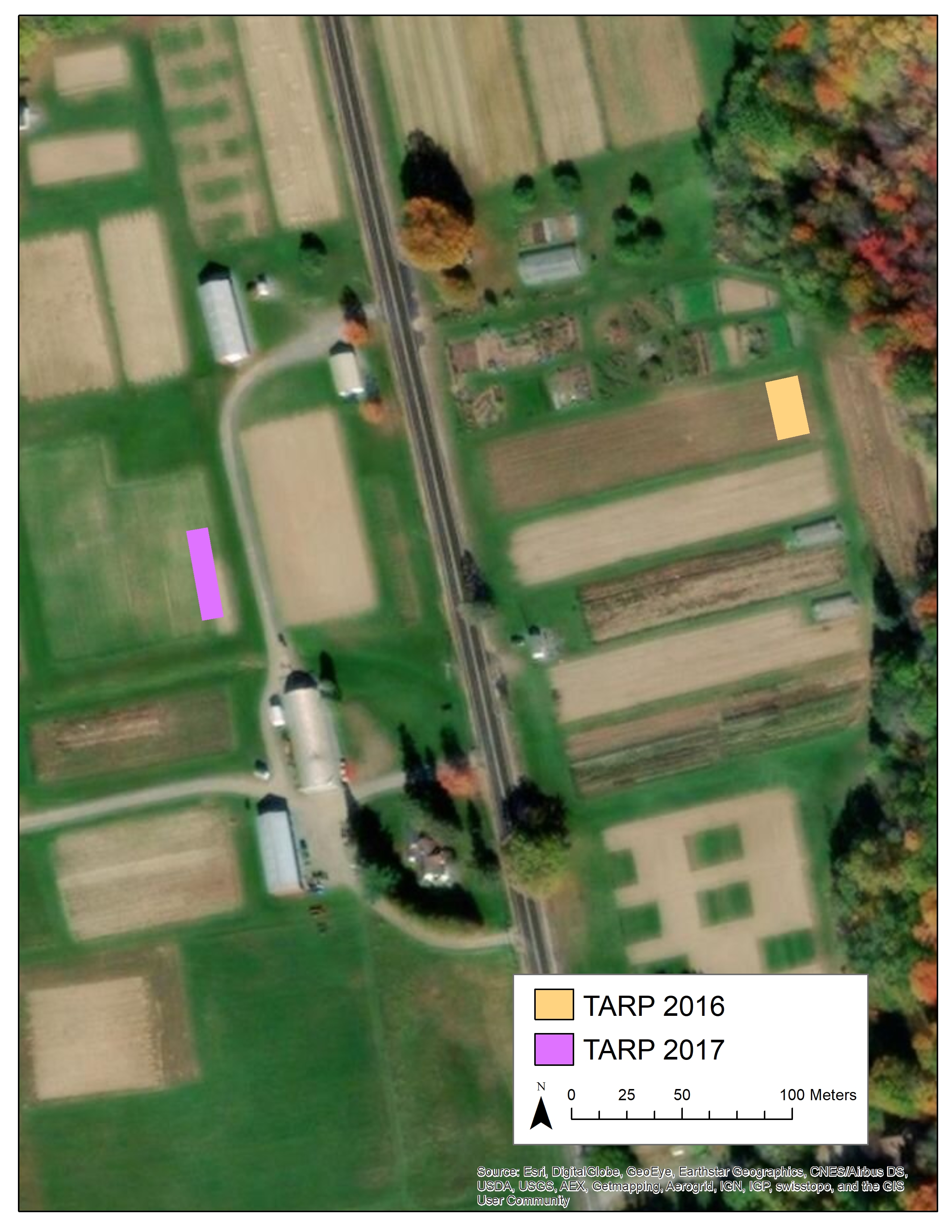
Figure A3. Aerial view showing locations of TARP experiments conducted during the summers of 2016 and 2017 to compare solarization and tarping.
